Editor’s note: If you’re looking for a quick way to start working with Swift 3.0 now, try out our apt-get ...
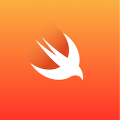
Editor’s note: If you’re looking for a quick way to start working with Swift 3.0 now, try out our apt-get ...
First things first, don’t call it the BeagleBone X15! This is a BeagleBoard. Affectionately named “The Beast” by its creators, ...
Update: If you don’t have Swift installed yet, head on over to our Ubuntu Packages page and get the latest ...
Editor’s Note: This article was written on December 6, 2015, days after Apple open-sourced Swift and made an Ubuntu distribution ...
Update! If you have the Swift compiler on your Linux box already, head on over to our tutorial on building ...
I had to create a new category named Hacking for this post. The end result is a Raspberry Pi outfitted ...
As I’ve said before, I’m a bit of a whore when it comes to learning new languages and development frameworks. ...
Editor’s note: This tutorial is written using Ubuntu Linux. If you are on a different platform you may need to ...