Let’s make use of the HiFive1 Rev B schematics to map out the GPIO controller device pins. Of particular interest ...
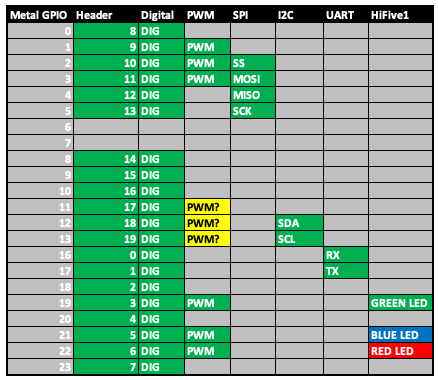
Let’s make use of the HiFive1 Rev B schematics to map out the GPIO controller device pins. Of particular interest ...
Today I’d like to introduce you to a new development board, the HiFive1 Rev B. Equipped with a RISC-V Freedom ...
Don’t ask me why I started looking at writing basic ARM assembly routines. Perhaps it’s for the thrill of it, ...